The Deadliest Flu: The Complete Story of the Discovery and Reconstruction of the 1918 Pandemic Virus
By Douglas Jordan with contributions from Dr. Terrence Tumpey and Barbara Jester
- Background: The deadly legacy of the 1918 pandemic and its importance for global efforts to prepare against future pandemic threats.
- Part 1 – Discovering a Lost Killer: The story of a virus hunter’s lifelong pursuit to discover the deadliest pandemic flu virus in human history.
- Part 2 – Building the Blueprint: The story of how a team of U.S. scientists decoded and assembled the genome of the 1918 virus.
- Part 3 – The Reconstruction: The story of how a CDC microbiologist reconstructed the live 1918 pandemic virus in a secure CDC laboratory to unravel its secrets and protect against future pandemics.
- Part 4 – Learning from the Past: How the world has progressed since the 1918 pandemic and the challenges posed by future pandemics.
- References
Background
The 100-year anniversary of the 1918 pandemic and the 10-year anniversary of the 2009 H1N1 pandemic are milestones that provide an opportunity to reflect on the groundbreaking work that led to the discovery, sequencing and reconstruction of the 1918 pandemic flu virus. This collaborative effort advanced understanding of the deadliest flu pandemic in modern history and has helped the global public health community prepare for contemporary pandemics, such as 2009 H1N1, as well as future pandemic threats.
The 1918 H1N1 flu pandemic, sometimes referred to as the “Spanish flu,” killed an estimated 50 million people worldwide, including an estimated 675,000 people in the United States.1,2,3,4 An unusual characteristic of this virus was the high death rate it caused among healthy adults 15 to 34 years of age.3 The pandemic lowered the average life expectancy in the United States by more than 12 years.3 A comparable death rate has not been observed during any of the known flu seasons or pandemics that have occurred either prior to or following the 1918 pandemic.3
The virus’ unique severity puzzled researchers for decades, and prompted several questions, such as “Why was the 1918 virus so deadly?”, “Where did the virus originate from?”, and “What can the public health community learn from the 1918 virus to better prepare for and defend against future pandemics?” These questions drove an expert group of researchers and virus hunters to search for the lost 1918 virus, sequence its genome, recreate the virus in a highly safe and regulated laboratory setting at CDC, and ultimately study its secrets to better prepare for future pandemics. The following is a historical recounting of these efforts, complete with references and descriptions of the contributions made by all of the remarkable men and women involved.
[Note: For a list of common questions and answers related to this work, see Q&A: Reconstruction of the 1918 Influenza Pandemic Virus.]
Discovering a lost killer
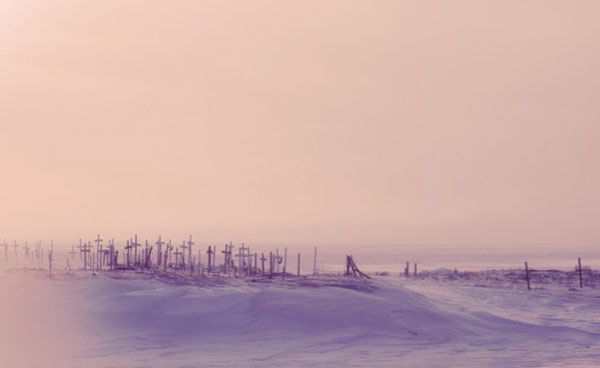
Site of the mass grave in Brevig Mission, Alaska, where 72 of the small village’s 80 adult inhabitants were buried after succumbing to the deadly 1918 pandemic virus. Photo credit: Angie Busch Alston.
For decades, the 1918 virus was lost to history, a relic of a time when the understanding of infectious pathogens and the tools to study them were still in their infancy. Following the 1918 pandemic, generations of scientists and public health experts were left with only the epidemiological evidence of the 1918 pandemic virus’ lethality and the deleterious impact it had on global populations. A small ocean-side village in Alaska called Brevig Mission would become both testament to this deadly legacy as well as crucial to the 1918 virus’ eventual discovery.
Today, fewer than 400 people live in Brevig Mission, but in the fall of 1918, around 80 adults lived there, mostly Inuit Natives. While different narratives exist as to how the 1918 virus came to reach the small village – whether by traders from a nearby city who traveled via dog-pulled sleds or even by a local mail delivery person – its impact on the village’s population is well documented. During the five-day period from November 15-20, 1918, the 1918 pandemic claimed the lives of 72 of the villages’ 80 adult inhabitants.
Later, at the order of the local government, a mass grave site marked only by small white crosses was created on a hill beside the village – a grim monument to a community all but erased from existence. The grave was frozen in permafrost and left untouched until 1951. That year, Johan Hultin, a 25-year-old Swedish microbiologist and Ph.D. student at the University of Iowa, set out on an expedition to Brevig Mission in the hopes of finding the 1918 virus and in the process unearth new insights and answers. Hultin believed that within that preserved burial ground he might still find traces of the 1918 virus itself, frozen in time within the tissues of the villagers whose lives it had claimed.
In 1951, Hultin successfully obtained permission from the village elders to excavate the Brevig Mission burial site. With the help of several of his university colleagues, Hultin set up a dig site over the grave. The excavation took days, as Hultin had to create campfires to thaw the earth enough to allow for digging. Two days in, Hultin came across the body of a little girl — her body was still preserved wearing a blue dress, and her hair was adorned with red ribbons5. Ultimately, Hultin successfully obtained lung tissue from four additional bodies buried at the site, but logistical and technological limitations of the time period would prove formidable.
In a conversation Hultin had decades later with CDC microbiologist Dr. Terrence Tumpey (see part III – the reconstruction), Hultin would explain how during the return trip from Alaska to the University of Iowa, he flew on a DC-3 propeller-driven airplane that was forced to make multiple stops along the trip to refuel. During each stop, Hultin – ever resourceful – would deboard the plane and attempt to re-freeze the lung samples using carbon dioxide from a fire extinguisher.
The noise generated from this activity apparently drew puzzled glances from fellow passengers and onlookers. Once back in Iowa, Hultin attempted to inject the lung tissue into chicken eggs to get the virus to grow.5 It did not. In the end, perhaps unsurprisingly, Hultin was unable to retrieve the 1918 virus from this initial attempt.
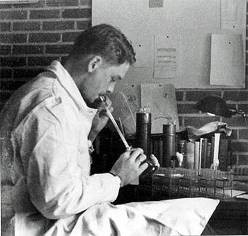
A picture of Johan Hultin working in the laboratory in 1951. Hultin’s initial attempt to rescue the 1918 virus was unsuccessful. Note: using one’s mouth to draw virus into a pipette is not considered a safe laboratory practice today. Laboratory safety practices have improved significantly in modern times. Photo credit: Johan Hultin.
It wouldn’t be until 46 years later, in 1997, that Hultin would have another opportunity to pursue the 1918 virus. That year, Hultin came across an article in the journal Science authored by Jeffery Taubenberger et al. entitled, “Initial Genetic Characterization of the 1918 “Spanish” Influenza Virus.”6 At the time, Dr. Taubenberger was a young molecular pathologist working for the Armed Forces Institute of Pathology in Washington, D.C.
In the article, Taubenberger and his team described their initial work to sequence part of the genome of the 1918 virus. The genome is the complete list of genetic instructions that make up an organism, similar to a blueprint used for construction. Many people are familiar with the concept of DNA, which is dual-stranded and determines the fundamental genetic characteristics of nearly all living things. However, the genome of an influenza virus consists of single-stranded RNA instead. Taubenberger’s team team successfully extracted RNA of the 1918 virus from lung tissue obtained from a 21-year-old male U.S. service member stationed in Fort Jackson, South Carolina. The serviceman had been admitted to the camp’s hospital on September 20, 1918, with a diagnosis of influenza infection and pneumonia. He died six days later on September 26, 1918, and a sample of his lung tissue was collected and preserved for later study.
From this tissue, Taubenberger’s group was able to sequence nine fragments of viral RNA from four of the virus’ eight gene segments. This work did not represent a complete sequence of the entire 1918 virus’ genome, but it provided a clearer picture of the pandemic virus than ever before. Based on the 1918 virus’ sequence data Taubenberger assembled in 1997, he and his fellow researchers initially claimed that the 1918 virus was a novel influenza A (H1N1) virus that belonged to a subgroup of viruses that came from humans and pigs, as opposed to birds.6 However, there was still much to learn about the virus.
After reading Taubenberger’s article, Hultin once again became inspired to attempt to recover the 1918 virus. Hultin wrote a letter to Taubenberger, asking if Taubenberger would be interested if he could return to Brevig Mission and obtain lung tissues from victims of the 1918 virus buried in the Alaskan permafrost. During a return phone call, Taubenberger responded, yes. A week later, Hultin departed for Brevig Mission once again with meager tools for the task. He famously borrowed his wife’s garden shears to assist in the excavation.
Forty-six years had passed since Hultin’s first trip to the gravesite, and he was now 72 years old. He once again sought permission to excavate the gravesite from the village council — which he obtained — and he also hired locals to assist in the work. Hultin paid for the trip himself at a personal cost of about $3,200.7 The excavation took about five days, but this time Hultin made a remarkable find.
Buried and preserved by the permafrost about 7 feet deep was the body of an Inuit woman that Hultin named “Lucy.” Lucy, Hultin would learn, was an obese woman who likely died in her mid-20s due to complications from the 1918 virus. Her lungs were perfectly frozen and preserved in the Alaskan permafrost. Hultin removed them, placed them in preserving fluid, and later shipped them separately to Taubenberger and his fellow researchers, including Dr. Ann Reid, at the Armed Forces Institute of Pathology.5 Ten days later, Hultin received a call from the scientists to confirm — to perhaps everyone’s collective astonishment — that positive 1918 virus genetic material had indeed been obtained from Lucy’s lung tissue.
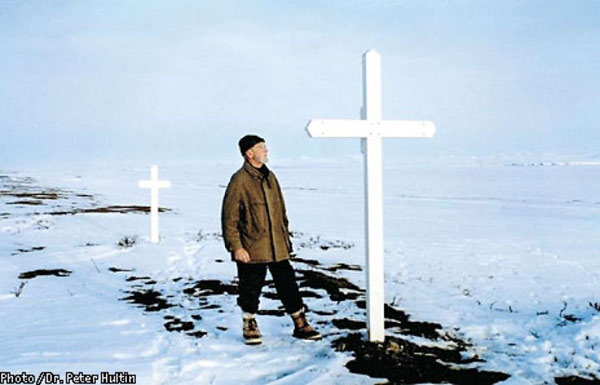
A picture of Johan Hultin at the Brevig Mission gravesite in 1997, 46 years after his first attempt to rescue the 1918 pandemic flu virus. Hultin saw that the small crosses that previously covered the site were missing, so Hultin built two large crosses (shown above) within the woodshop of a local school to mark the gravesite. Photo credit: Johan Hultin.
Building the Blueprint
The initial impact of this discovery would first be described in a February 1999 paper in the Proceedings of the National Academy of Science (PNAS) journal entitled “Origin and evolution of the 1918 “Spanish” influenza virus hemagglutinin gene,” by Ann Reid et al. 8 Hultin was acknowledged as a co-author. In the paper, the authors described their effort to sequence (i.e., characterize) the 1918 virus’s hemagglutinin “HA” gene.
The HA gene of an influenza virus determines the properties of the virus’s HA surface proteins. These HA surface proteins allow an influenza virus to enter and infect a healthy respiratory tract cell. HA is also targeted by antibodies produced by the immune system to fight infection. Modern flu vaccines work by targeting an influenza virus’ unique HA (a fact that virologist Dr. Peter Palese, featured later in this article, helped pioneer).
In the 1999 study, the authors succeeded in sequencing the full length HA gene sequence of the 1918 virus. To accomplish this, the authors used RNA fragments of the virus obtained from the bodies of the formerly described 21-year-old Fort Jackson service member, “Lucy” from Brevik Mission, and a third person, a 30-year-old male service member stationed at Camp Upton, New York. This man was admitted to the camp hospital with influenza on September 23, 1918, had a rapid clinical course of illness, and died from acute respiratory failure on September 26, 1918.
Sequencing results suggested that the ancestor of the 1918 virus infected humans sometime between 1900 and 1915. Drs. Reid and Taubenberger noted that the 1918 HA gene had a number of mammalian as opposed to avian adaptations, and was more human-like or swine-like depending on the method of analysis. Phylogenetic analysis, which is used to group influenza viruses in accordance with their evolutionary development and diversity, placed the 1918 virus’ HA within and around the root of the mammalian clade. This means that it likely was an ancestor or closely related to the earliest influenza viruses known to infect mammals. However, the authors believed the virus likely obtained its HA from avian viruses, but were unsure how long the virus may have been adapting in a mammalian host before emerging in pandemic form.
According to the authors, the existing strain to which the 1918 virus sequences were most closely related was “A/sw/Iowa/30,” the oldest classical swine influenza strain. The authors noted that contemporary avian influenza virus strains are very different from the 1918 pandemic virus, and unfortunately older avian strains from around the time of the 1918 pandemic were not available for study. The authors also noted that the 1918 virus’ HA1 had only four glycosylation sites, which is different from modern human HA’s which have accumulated up to five additional glycosylation sites through the process of antigenic drift. Antigenic drift refers to small changes in the genes of influenza viruses that happen continually over times as the virus copies itself. Antigenic drift is one reason why there is a flu season every year and also a reason for why people can get the flu multiple times in their lifetime.
Glycosylation sites are believed to be necessary for the function of influenza viruses, and the inclusion of additional glycosylation sites is believed to be an adaptation of the virus to human hosts. Also of note, the authors did not see any genetic changes in the 1918 virus’ HA that would explain its exceptional virulence.
Unlike modern virulent avian influenza strains, such as avian influenza A (H5) and (H7) viruses, the 1918 virus’ HA did not possess a “cleavage site” mutation, which is a recognized genetic marker for virulence, i.e., the severity or harmfulness of a disease. The insertion of amino acids in the HA cleavage site can allow an influenza virus to grow in tissues outside of its normal host cells. In the absence of such obvious markers, Dr. Reid and her fellow researchers concluded that there were likely multiple genetic factors responsible for the 1918 virus’ severity.
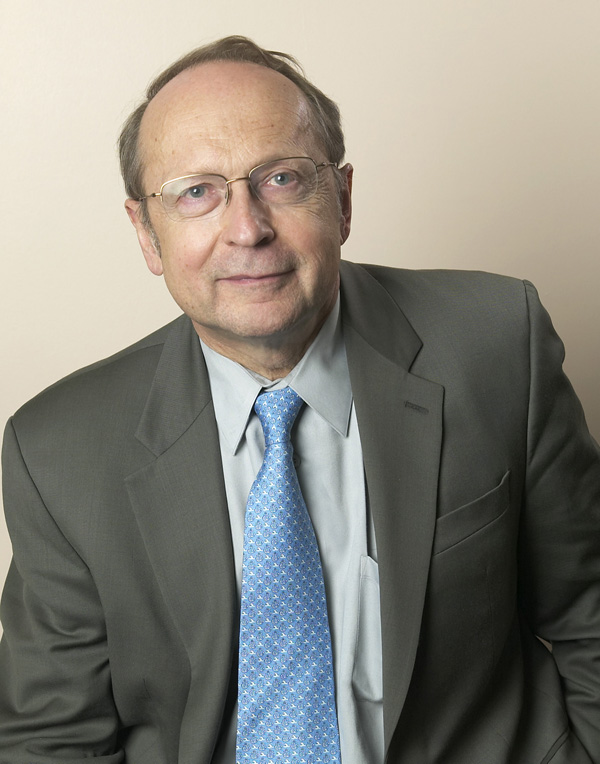
Microbiologist Dr. Peter Palese and his team created the plasmids used by Dr. Terrence Tumpey to reconstruct the 1918 pandemic virus. Palese has many accomplishments, including creating the first genetic maps of influenza A, B, and C viruses, as well as defining the mechanism used by the majority of current influenza antiviral drugs. Photo credit: Wikipedia (https://en.wikipedia.org/wiki/Peter_Palese)
A follow-up paper published in June 2000, entitled “Characterization of the 1918 “Spanish” Influenza Virus Neuraminidase Gene,” described sequencing of the 1918 virus’ neuraminidase (NA) gene.9 In an influenza virus, the neuraminidase gene is responsible for coding the virus’ NA surface proteins (see prior virus image for reference). An influenza virus’ NA surface proteins allow an influenza virus to escape an infected cell and infect other cells. Therefore, it plays an important role in spreading influenza infection. The author noted that NA is also targeted by the immune system, and that antibodies against NA do not prevent infection, but they do significantly limit the ability of the virus to spread.
Of note, the authors were able to sequence the entire code of the 1918 virus’ NA from the virus sample obtained from “Lucy’s” body. So here again, Hultin’s work proved invaluable. The authors found that the NA gene of the 1918 virus shared many sequence and structural characteristics with both mammalian and avian influenza virus strains.9 Phylogenetic analysis suggested the NA gene of the 1918 virus was intermediately located between mammals and birds, suggesting that it likely was introduced into mammals shortly before the 1918 pandemic. Furthermore, the 1918 virus’ NA obtained from Lucy suggested that it is very similar to the ancestor of all subsequent swine and human isolates.9
Overall, the phylogenetic analysis seemed to indicate that the ultimate source of the 1918 virus’ NA was avian in nature, but the authors couldn’t determine the pathway from its avian source to the virus’ final pandemic form. Regarding genetic features of the NA that could explain the 1918 virus’ severity, the researchers were once again unable to find any single feature of the 1918 NA that contributed to the virus’ virulence.9 For example, in some modern influenza viruses, the loss of a glycosylation site in NA at amino acid 146 (in WSN/33) contributes to virulence and also results in the virus attacking the nervous system in mice. However, this change was not found in the NA of the 1918 virus.
Following this study, a series of additional studies were published, each detailing the findings from each of the 1918 virus’ remaining genes (flu viruses have 8 genes in total). In 2001, a paper by Christopher Basler et al. published in the Proceedings of the National Academic of Science (PNAS) described the sequencing of the 1918 virus’ nonstructural (NS) gene.10 A 2002 study in the Journal of Virology by Ann Reid et al. described sequencing of the virus’ matrix gene.11 Two years later, a 2004 Journal of Virology study described the sequencing of the 1918 virus’ nucleoprotein (NP) gene.12 In 2005, the virus’ polymerase genes were sequenced by Taubenberger et al and described in a Nature article.13 This final study bookended the nearly decade long process of sequencing the entire genome of the 1918 virus.
With the entire genome of the 1918 virus now sequenced, the necessary information was in place to reconstruct a live version of the 1918 virus. However, one more intermediate step was needed to start the reverse genetics process, which was to create plasmids for each of the 1918 virus’ eight gene segments.
This task was undertaken by renowned microbiologist, Dr. Peter Palese and Dr. Adolfo Garcia-Sastre at Mount Sinai School of Medicine in New York City. A plasmid is a small circular DNA strand that can be amplified (or replicated) in the laboratory. Years earlier, Dr. Palese helped pioneer the use of plasmids in reverse genetics to produce viable influenza viruses. The techniques he developed allowed the relationships between the structure and function of viral genes to be studied, and these efforts paved the way for the techniques used to reconstruct the 1918 virus. Once Dr. Palese and his colleagues at Mount Sinai completed creation of the plasmids, they were shipped to CDC so the official process of reconstruction could begin.
The Reconstruction
The decision to reconstruct the deadliest pandemic flu virus of the 20th century was made with considerable care and attention to safety. Senior government officials decided on CDC headquarters in Atlanta as the location of the reconstruction. CDC conducted two tiers of approvals: first by CDC’s Institutional Biosafety Committee and the second by CDC’s Institutional Animal Care and Use Committee, before work in the laboratory began. The work would be performed using stringent biosafety and biosecurity precautions and facilities, including what’s known as Biosecurity Level 3 (BSL-3) practices and facilities with enhancements.
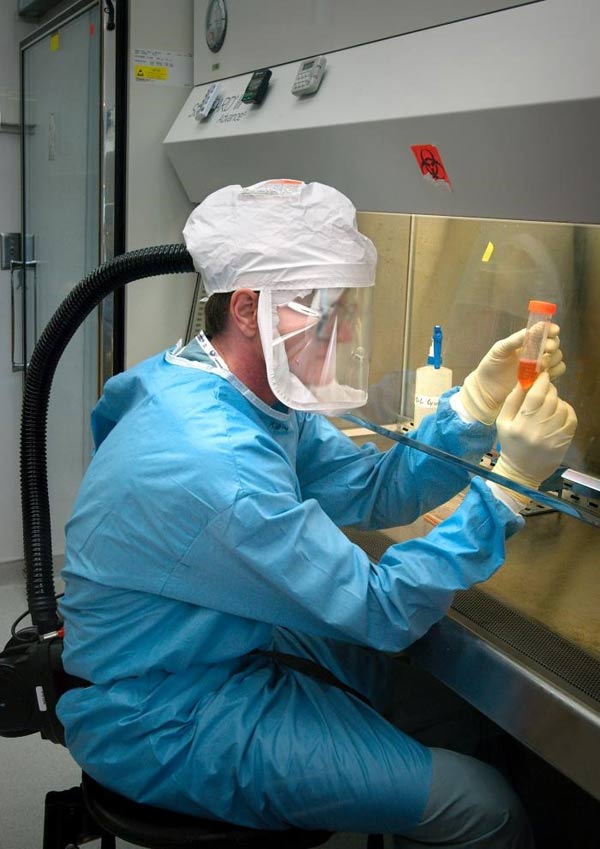
A picture of Dr. Terrence Tumpey working in BSL3 enhanced laboratory conditions. This includes (but isn’t limited to) use of a powered air purifying respirator (PAPR), double gloves, suit, and working within a Class II biosafety cabinet (BSC). Today, Dr. Tumpey is the branch chief of the Immunology and Pathogenesis Branch in CDC’s Influenza Division. Photo credit: James Gathany - Public Health Image Library #7989.
For reference, there are four biosafety levels that correspond to the degree of risk posed by research, with 1 posing the least risk and 4 posing the greatest risk. Each biosecurity level also corresponds with specific laboratory practices and techniques, personnel training requirements, laboratory equipment, and laboratory facilities that are appropriate for the operations being performed. The stringency of these considerations – again ranging from 1 as the lowest to 4 as the highest — is designed to protect the personnel performing the work, the environment and the community.
Each biosecurity level includes considerations for what is known as “primary” and “secondary” barriers. Examples of primary barriers include safety cabinets, isolation chambers, gloves and gowns, whereas secondary barriers include considerations such as the construction of the facility and HEPA filtration of air in the laboratory. The specific criteria for each biosafety level are detailed in the CDC/NIH publication Biosafety in Microbiological and Biomedical Laboratories.
A BSL3 laboratory with enhancements includes a number of primary and secondary barriers and other considerations. For example, all personnel must wear a powered air purifying respirator (PAPR), double gloves, scrubs, shoe covers and a surgical gown. They also must shower before exiting the laboratory. In addition, all work with the virus or animals must be conducted within a certified Class II biosafety cabinet (BSC), and airflow within the laboratory is directionally controlled and filtered so that it cannot accidentally exit the laboratory.
For the reconstruction of the 1918 virus, additional rules were created to govern the experiments to be conducted. For example, to prevent mix-ups and cross-contamination, work on the 1918 virus could not take place alongside work on other influenza viruses.
As part of security and safety considerations, CDC’s Office of the Director determined that only one person would be granted permission, laboratory access, and the tremendous responsibility of reconstructing the 1918 virus. That person was trained microbiologist Dr. Terrence Tumpey, who was approved for the project by then CDC director, Dr. Julie Gerberding. Reconstruction of the 1918 virus also was approved by the National Institute of Allergy and Infectious Disease (NIAID) within the National Institutes of Health (NIH), which partially funded the project.
Dr. Tumpey was formerly a U.S. Department of Agriculture microbiologist at the Southeast Poultry Research Laboratory in Athens, Georgia. Earlier in his career, he had applied for an American Society of Microbiology (ASM) postdoctoral fellowship with CDC microbiologist and flu expert Dr. Jacqueline Katz, who recently retired as Deputy Director of CDC’s Influenza Division. This two-year fellowship in CDC’s Influenza Division would mark the beginning of Dr. Tumpey’s career at CDC. He officially transferred employment to CDC for the purpose of studying human health implications of influenza viruses, including the 1918 pandemic virus.
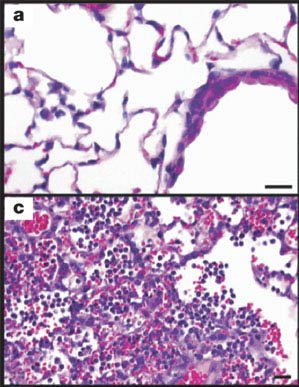
The 1918 virus was extremely virulent. Image a) shows mouse lung tissue infected with a human seasonal H1N1 flu virus. Image c) shows the impact of the 1918 virus in mouse lung tissue. The 1918 virus replicates quickly and causes severe disease in the lung tissues of mice. In 1918, the virus caused severe disease in the lungs of people infected, as well. Photo credit: CDC, Science.
Dr. Tumpey’s work to reconstruct the complete 1918 virus began in the summer of 2005. To reduce risk to colleagues and the public, he was required to work on the virus alone and only after hours when fellow colleagues had exited the laboratories for the day and gone home. A biometric fingerprint scan was required for access into the BSL-3E laboratory, and the virus storage freezers were only accessible via an iris scan of his eyes. Dr. Tumpey was required to take a prescribed prophylactic (preventative) daily dose of the influenza antiviral drug, oseltamivir, as an additional safety precaution to prevent him from becoming infected. Should he become infected, he was informed that he would be placed in quarantine and denied contact with the outside world. He understood and accepted this responsibility and its consequences.
Using reverse genetics, Dr. Tumpey took the plasmids created by Dr. Palese for each of the 1918 virus’ eight gene segments and inserted them into human kidney cells. The plasmids then instructed the cells to reconstruct the RNA of the complete 1918 virus. For multiple weeks in July 2005, colleagues and collaborators asked Dr. Tumpey if he had the 1918 virus and if it had appeared in cell-culture yet.
On the day the 1918 virus appeared in his cell-culture, Dr. Tumpey knew history had been made, and in fact, a historic virus had been brought back from extinction. He sent a playful, Neil Armstrong-inspired email later that day to colleagues and collaborators, which simply said “That’s one small step for man, one giant leap for mankind.” Everyone then knew what had been accomplished. Dr. Tumpey had become the first man to reconstruct the complete 1918 virus. The next step was to study it and unlock its deadly secrets.
Laboratory studies on the reconstructed 1918 virus began in August 2005. A report of this work, “Characterization of the Reconstructed 1918 Spanish Influenza Pandemic Virus”, was published in the October 7, 2005, issue of Science.14 To evaluate the 1918 virus’ pathogenicity (i.e., the ability of the virus to cause disease and harm a host), animal studies involving mice were conducted. The mice were infected with the 1918 virus, and measures of morbidity (i.e., weight loss, virus replication, and 50% lethal dose titers) were collected and documented. For comparison, other mice were infected with different influenza viruses that were designed via reverse genetics to have varying combinations of genes from the 1918 virus and contemporary human seasonal influenza A(H1N1) viruses. These viruses are called “recombinant viruses.”
The fully reconstructed 1918 virus was striking in terms of its ability to quickly replicate, i.e., make copies of itself and spread infection in the lungs of infected mice. For example, four days after infection, the amount of 1918 virus found in the lung tissue of infected mice was 39,000 times higher than that produced by one of the comparison recombinant flu viruses.14
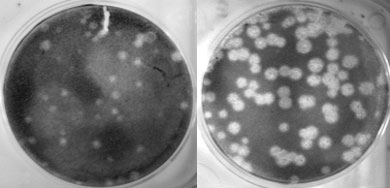
The left picture shows replication of a human seasonal flu virus called Tx/91 in cell culture. The picture on the right shows how when the polymerase (PB1) gene of this same virus is exchanged with that of the 1918 virus, the resulting virus’ ability to replicate (i.e., make copies of itself) is greatly enhanced. Photo credit: Terrence Tumpey, CDC.
Furthermore, the 1918 virus was highly lethal in the mice. Some mice died within three days of infection with the 1918 virus, and the mice lost up to 13% of their body weight within two days of infection with the 1918 virus. The 1918 virus was at least 100 times more lethal than one of the other recombinant viruses tested.14 Experiments indicated that 1918 virus’ HA gene played a large role in its severity. When the HA gene of the 1918 virus was swapped with that of a contemporary human seasonal influenza A (H1N1) flu virus known as “A/Texas/36/91” or Tx/91 for short, and combined with the remaining seven genes of the 1918 virus, the resulting recombinant virus notably did not kill infected mice and did not result in significant weight loss.14
Other experiments were conducted to determine if infection with the 1918 virus could spread to other vital organs of mice — such as the brain, heart, liver and spleen. Laboratory testing did not detect virus in these organs, suggesting that the 1918 virus did not cause systemic infection in its victims.
However, one well-documented effect of the 1918 virus was rapid and severe lung damage. In 1918, victims of the pandemic virus experienced fluid-filled lungs, as well as severe pneumonia and lung tissue inflammation. Within four days post infection, mice infected with the 1918 virus experienced similar lung complications, suggesting that this was a unique aspect of the 1918 virus’ severity.14
The impact of the 1918 virus on lung tissue was also studied using a human lung cell line (known as Calu-3 cells). The amount of 1918 flu virus was measured in the cells at 12, 16 and 24 hours post infection and these results were compared to those produced by recombinant viruses with a combination of genes from the 1918 virus mixed with genes from contemporary human seasonal flu viruses. Similar to the experiments involving mice, the 1918 virus quickly multiplied and spread within the human lung cells. So much so, that the 1918 virus produced as much as 50 times the amount of virus in human lung cells as one of the comparison viruses. These experiments suggested that in addition to the HA, the polymerase genes of the 1918 virus played a significant role in the virus’ infectivity and virulence in human lung tissue.14
Another set of experiments was conducted to better understand the possible avian origins of the 1918 virus. The earlier sequencing efforts led by Dr. Taubenberger and Reid had suggested that the 1918 virus’ gene segments were more closely related to avian influenza A(H1N1) viruses than H1N1 viruses found in other mammals. Researchers were interested to know whether the 1918 virus would be lethal to fertilized chicken eggs, i.e., chicken eggs containing an embryo, similar to modern highly pathogenic avian influenza viruses.
To find an answer, 10-day old fertilized chicken eggs were inoculated with the 1918 virus. The 1918 virus proved lethal for the chicken egg embryos, similar to the effects caused by contemporary H1N1 bird flu viruses.14 Notably, comparison experiments using human seasonal influenza A(H1N1) viruses did not have this destructive effect on chicken embryos. Furthermore, the recombinant flu viruses that Dr. Tumpey created containing two, five or seven genes of the 1918 virus also did not hurt chicken embryos.14 Similar to the results of the studies conducted in mice and human lung cell, these fertilized chicken egg experiments indicated that the HA and polymerase genes of the 1918 virus both likely played roles in its virulence.
The work conducted by Dr. Tumpey and his CDC colleagues provided new information about the properties that contributed to the virulence of the 1918 virus. Dr. Tumpey determined that the HA and PB1 virus genes of the virus played particularly important roles in its infectiousness and severity. However, as his experiments involving recombinant flu viruses with some but not all of the 1918 virus’s genes showed, it was not any single component of the 1918 virus but instead the unique combination of all of its genes together that made it so particularly dangerous.
Tumpey and colleagues wrote “the constellation of all eight genes together make an exceptionally virulent virus.”14 No other human influenza viruses tested were as exceptionally virulent. In that way, the 1918 virus was special – a uniquely deadly product of nature, evolution and the intermingling of people and animals. It would serve as a portent of nature’s ability to produce future pandemics of varying public health concern and origin.
Learning from the Past
Since 1918, the world has experienced three additional pandemics, in 1957, 1968, and most recently in 2009. These subsequent pandemics were less severe and caused considerably lower mortality rates than the 1918 pandemic.2,3,4 The 1957 H2N2 pandemic and the 1968 H3N2 pandemic each resulted in an estimated 1 million global deaths, while the 2009 H1N1 pandemic resulted in fewer than 0.3 million deaths in its first year.3,4 This perhaps begs the question of whether a high severity pandemic on the scale of 1918 could occur in modern times.
Many experts think so. One virus in particular has garnered international attention and concern: the avian influenza A(H7N9) virus from China. The H7N9 virus has so far caused 1,568 human infections in China with a case-fatality proportion of about 39% since 2013. However, it has not gained the capability to spread quickly and efficiently between people. If it did, experts believe it could result in a pandemic with severity comparable to the 1918 pandemic. So far, it has shown only limited ability to spread between people. Most human infections with this virus have result from exposure to birds.
When considering the potential for a modern era high severity pandemic, it is important; however, to reflect on the considerable medical, scientific and societal advancements that have occurred since 1918, while recognizing that there are a number of ways that global preparations for the next pandemic still warrant improvement.
Besides the properties of the virus itself, many additional factors contributed to the virulence of the 1918 pandemic. In 1918, the world was still engaged in World War I. Movement and mobilization of troops placed large numbers of people in close contact and living spaces were overcrowded. Health services were limited, and up to 30% of U.S. physicians were deployed to military service.3
In addition, medical technology and countermeasures at the time were limited or non-existent. No diagnostic tests existed at the time that could test for influenza infection. In fact, doctors didn’t know influenza viruses existed. Many health experts at the time thought the 1918 pandemic was caused by a bacterium called “Pfeiffer’s bacillus,” which is now known as Haemophilus influenzae.
Influenza vaccines did not exist at the time, and even antibiotics had not been developed yet. For example, penicillin was not discovered until 1928. Likewise, no flu antiviral drugs were available. Critical care measures, such as intensive care support and mechanical ventilation also were not available in 1918.4 Without these medical countermeasures and treatment capabilities, doctors were left with few treatment options other than supportive care.3
In terms of national, state and local pandemic planning, no coordinated pandemic plans existed in 1918. Some cities managed to implement community mitigation measures, such as closing schools, banning public gatherings, and issuing isolation or quarantine orders, but the federal government had no centralized role in helping to plan or initiate these interventions during the 1918 pandemic.3
Today, considerable advancements have been made in the areas of health technology, disease surveillance, medical care, medicines and drugs, vaccines and pandemic planning. Flu vaccines are now produced and updated yearly, and yearly vaccination is recommended for everyone 6 months of age and older. Antiviral drugs now exist that treat flu illness, and in the event of virus exposure, can be used for prophylaxis (prevention), as well. Importantly, many different antibiotics are now available that can be used to treat secondary bacterial infections.
Diagnostic tests for identifying influenza are now available and they are improving over time. Current rapid tests for flu, also known as RIDTs, provide results within 15 minutes and have sensitivities ranging from 50-70%. Recently, new “rapid molecular assays” have become available that are timely and much more accurate than RIDTs. Just as important as these advancements in diagnostic tests are the improvements that have been made in laboratory testing capacity both within the United States and globally.
The World Health Organization (WHO)’s Global Influenza Surveillance and Response System (GISRS) is a global flu surveillance network that monitors changes in seasonal flu viruses and also monitors the emergence of novel (i.e., new in humans) flu viruses, many of which originate from animal populations. Through animal and human interactions and environmental exposures, these viruses can cause human infections. CDC in Atlanta is one of WHO’s six Collaborating Centers for Reference and Research on Influenza (joining others in Australia, China, Japan and the United Kingdom). The WHO collaborating centers collect influenza viruses obtained from respiratory specimens from patients around the world, and they are supported by 143 National Influenza Centers in 114 WHO member countries.3
Expanding laboratory testing and flu surveillance capacity around the world has been an important focus of pandemic preparedness efforts. In 2004, CDC began an international surveillance capacity building initiative that entailed a 5-year period of financial support to improve laboratory diagnostic tests and surveillance of influenza like illness (ILI) and severe acute respiratory infection (SARI) in 39 partner countries.
In 2008, CDC established the International Reagent Resource (IRR), which provides reagents to laboratories around the world to identify seasonal influenza A and B viruses, as well as novel influenza A viruses. During the 2009 H1N1 pandemic, the IRR distributed a new CDC developed 2009 H1N1 PCR assay to domestic public health laboratories and laboratories around the world less than 2 weeks after the 2009 H1N1 virus was first identified. This considerably enhanced the ability of the global flu surveillance community to track spread of the virus.3
As part of WHO’s International Health Regulations (IHR), countries must notify WHO within 24 hours of any case of human infection caused by a novel influenza A virus subtype. This requirement is designed to help quickly identify emerging viruses with pandemic potential.
Since 2010, CDC has used its Influenza Risk Assessment Tool (IRAT) to evaluate and score emerging novel influenza A viruses and other viruses of potential public health concern. The score provided by the IRAT answers two questions: 1) What is the risk that a virus that is novel in humans could result in sustained human to human transmission? and: 2) What is the potential for the virus to substantially impact public health if it does gain the ability to spread efficiently from person to person? Results from the IRAT have helped public health experts target pandemic preparedness resources against the greatest disease threats and to prioritize the selection of candidate vaccine viruses and the development of pre-pandemic vaccines against emergent viruses with the greatest potential to cause a severe pandemic.
When pre-pandemic vaccines are made, they are stored in the Strategic National Stockpile, along with facemasks, antiviral drugs and other materials that can be used in case of a pandemic.
All of these resources, tools, technologies, programs and activities are excellent tools for pandemic planning, and pandemic planning itself has improved significantly since 1918. In the United States, the Department of Health and Human Services (HHS) maintains a national Pandemic Influenza Plan, and this plan was updated in 2017. The World Health Organization (WHO) has published instructions for countries to use in developing their own national pandemic plans, as well as a checklist for pandemic influenza risk and impact management.3
Planners have access to other materials as well. For example, in 2014, CDC published a pandemic framework with six intervals that fall within a pandemic curve. Each interval helps with prioritizing data collection, government resources and interventions, and other important activities during the pandemic. In addition, CDC experts have devised a Pandemic Severity Assessment Framework that uses data to assign severity and transmissibility scores to pandemics. The tool is useful for planning purposes and for determining appropriate mitigations based on the severity of a pandemic. In addition, guidelines for non-pharmaceutical interventions, such as closing schools and large social gatherings, have been established and revised, for use during a pandemic.
While all of these plans, resources, products and improvements show that significant progress has been made since 1918, gaps remain, and a severe pandemic could still be devastating to populations globally. In 1918, the world population was 1.8 billion people. One hundred years later, the world population has grown to 7.6 billion people in 2018.3 As human populations have risen, so have swine and poultry populations as a means to feed them. This expanded number of hosts provides increased opportunities for novel influenza viruses from birds and pigs to spread, evolve and infect people. Global movement of people and goods also has increased, allowing the latest disease threat to be an international plane flight away. Due to the mobility and expansion of human populations, even once exotic pathogens, like Ebola, which previously affected only people living in remote villages of the African jungle, now have managed to find their way into urban areas, causing large outbreaks.
If a severe pandemic, such as occurred in 1918 happened today, it would still likely overwhelm health care infrastructure, both in the United States and across the world. Hospitals and doctors’ offices would struggle to meet demand from the number of patients requiring care. Such an event would require significant increases in the manufacture, distribution and supply of medications, products and life-saving medical equipment, such as mechanical ventilators. Businesses and schools would struggle to function, and even basic services like trash pickup and waste removal could be impacted.
The best defense against the flu continues to be a flu vaccine, but even today, flu vaccines face a number of challenges. One challenge is that flu vaccines are often moderately effective, even when well matched to circulating viruses. But perhaps the biggest challenge is the time required to manufacture a new vaccine against an emerging pandemic threat. Generally, it has taken about 20 weeks to select and manufacture a new vaccine.
During the 2009 H1N1 pandemic, the first doses of pandemic vaccine did not become available until 26 weeks after the decision to manufacture a monovalent vaccine.3 As a result, most vaccinations in the United States occurred after the peak of 2009 H1N1 illness. The HHS Pandemic Influenza Plan has a goal of reducing the timeframe to make a pandemic flu vaccine from 20 weeks to 12 weeks, but accomplishing this is challenging.
One possible solution is to create more broadly protective and longer lasting vaccines. Creation of a “universal vaccine” continues to elude the world’s top scientists, but in the future, it could become a reality. In the meantime, health officials seek to get the most out of new and existing flu vaccine technologies, such as cell based and recombinant vaccines, which are not reliant on a supply of chicken eggs, like traditional vaccines, and have the potential to be produced faster.
One other vaccine issue is the inadequate global capacity for mass producing flu vaccines. Global pandemic flu vaccine capacity was estimated to be 6.4 billion doses in 2015, but this is not enough to cover even half of the world’s population, should two doses of a pandemic vaccine be required for protection.3
Other challenges at a global level include surveillance capacity, infrastructure and pandemic planning. The majority of counties that report to the WHO still do not have a national pandemic plan, and critical and clinical care capacity, especially in low income countries, continues to be inadequate to the demands of a severe pandemic.3 In 2005, milestones were created in the revised International Health Regulations (IHR) for countries to improve their response capacity for public health emergencies, but in 2016, only one-third of countries were in compliance.3
All of these issues show that more work needs to be done, both here in the United States and internationally, to prepare for the next pandemic. On May 7, 2018, The Rollins School of Public Health at Emory University in partnership with the U.S. Centers for Disease Control and Prevention, hosted a one-day symposium on the 100-year anniversary of the 1918 influenza pandemic. The event involved experts from government and academia discussing current pandemic threats and the future of pandemic preparedness, influenza prevention and control. U.S. and global influenza experts who attended the meeting agreed that we still face great challenges to prepare for future flu pandemics, but part of the solution is recognizing these challenges and working together with the rest of the world to address them.
For more information on the 1918 pandemic, see CDC’s 1918 (H1N1 virus) website. For more information about influenza pandemics, see Pandemic Influenza.
References
- P. Johnson and J. Mueller. Updating the accounts: global mortality of the 1918–1920 “Spanish” influenza pandemic. Bull. Hist. Med. 2002. 76(1): 105-115.
- Barbara Jester et al. Historical and clinical aspects of the 1918 H1N1 pandemic in the United States. Virology 2019. 527: 32-37.
- Barbara Jester et al. Readiness for Responding to a Severe Pandemic 100 Years After 1918. Am J Epidemiol. July 2018. 187(12): 2596-2602. DOI: 10.1093/aje/kwy165.
- Barbara Jester et al. 100 Years of Medical Countermeasures and Pandemic Influenza Preparedness. Am J Public Health. 2018; 108: 1469-1472. Doi:10.2105/AJPH.2018.304586.
- NYT Magazine: Why revive a deadly flu virus? https://www.nytimes.com/2006/01/29/magazine/why-revive-a-deadly-flu-virus.html.
- K. Taubenberger, Ann Reid, Amy Krafft et al. Initial Genetic Characterization of the 1998 “Spanish’ Influenza Virus. Science. Mar 1997; Vol 275, Issue 5307, pp 1793-1796.
- San Francisco Chronicle: The Virus detective / Dr. John Hultin has found evidence of the 1918 flu epidemic that had eluded experts for decades https://www.sfgate.com/magazine/article/The-Virus-detective-Dr-John-Hultin-has-found-2872017.php
- Ann Reid et al. Origin and evolution of the 1918 “Spanish” influenza virus hemagglutinin gene PNAS. February 1999. Vol 96. Pp. 1651-1656.
- Ann Reid et al. Characterization of the 1918 “Spanish Influenza Virus Neuraminidase Gene. PNAS. Vol. 97. No. 12. June 2000. Pp. 6785-6790.
- Christopher Basler et al. “Sequence of the 1918 pandemic influenza virus nonstructural gene (NS) segment and characterization of recombinant viruses bearing the 1918 NS genes” PNAS. Vol. 98. No. 5. February 2001 Pp. 1746-2751.
- Ann Reid et al. “Characterization of the 1918 “Spanish” Influenza Virus Matrix Gene Segment” Journal of Virology. Nov 2002 Vol. 76. No. 21. pp. 10717-10723.
- Ann Reid et al. “Novel Origin of the 1918 Pandemic Influenza Virus Nucleoprotein Gene” Journal of Virology. Nov 2004. Vol. 78, No. 22. Pp. 12462-12470.
- Jeffery K. Taubenberger et al. “Characterization of the 1918 influenza virus polymerase genes” Nature. October 2005. Vol 437 (6). Pp. 889-893.
- Tumpey TM, Baster CF, Aguilar PV, et al. Characterization of the Reconstructed 1918 Spanish Influenza Pandemic Virus” Science. October 2005. 310(5745): 77-80.
Related Resources
- Terrence Tumpey et al. “Existing antivirals are effective against influenza viruses with genes from the 1918 pandemic virus.” PNAS. October 2002. Vol. 99. No. 21 pp. 13849-13854.
- Terrence Tumpey et al. “Pathogenicity and immunogenicity of influenza viruses with genes from the 1918 pandemic virus. PNAS. March 2004. Vol. 101 No. 9. Pp. 3166-3171.
- Gibbs MJ, Armstrong JS, Gibbs AJ, et al. “Recombination in the Hemagglutinin Gene of the 1918 “Spanish Flu” Science. September 2001. Vol 293 (5536). Pp. 1842-1845.
- Laurel Glaser et al. “A Single Amino Acid Substitution in 1918 Influenza Virus Hemagglutinin Changes Receptor Binding Specificity” Journal of Virology. Sept 2005. Vol 79. No. 17. Pp. 11533-11536.
- Ervin Fodor et al. “Rescue of Influenza A Virus from Recombinant DNA” Journal of Virology. Nov 1999. Vol 73. No. 11. Pp. 9679-9682.
- Darwyn Kobasa et al. “Enhanced virulence of influenza A viruses with the haemagglutinin of the 1918 pandemic virus.” Nature. October 2004. Vol 431 pp. 703-707.
- Masato Hatta et al. “Molecular Basis for High Virulence of Hong Kong H5N1 Influenza A Viruses” Science. September 2001. Vol 293. Pp. 1840-1842.
- Peter Palese Bio – Icahn School of Medicine at Mount Sinai: http://labs.icahn.mssm.edu/paleselab/